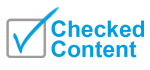
Rutherfordium
Background to the schools Wikipedia
This Schools selection was originally chosen by SOS Children for schools in the developing world without internet access. It is available as a intranet download. Before you decide about sponsoring a child, why not learn about different sponsorship charities first?
Rutherfordium | ||||||||||||||||||||||||||||||||||||||||||||||||||||||||||||
---|---|---|---|---|---|---|---|---|---|---|---|---|---|---|---|---|---|---|---|---|---|---|---|---|---|---|---|---|---|---|---|---|---|---|---|---|---|---|---|---|---|---|---|---|---|---|---|---|---|---|---|---|---|---|---|---|---|---|---|---|
104Rf
|
||||||||||||||||||||||||||||||||||||||||||||||||||||||||||||
|
||||||||||||||||||||||||||||||||||||||||||||||||||||||||||||
Appearance | ||||||||||||||||||||||||||||||||||||||||||||||||||||||||||||
unknown | ||||||||||||||||||||||||||||||||||||||||||||||||||||||||||||
General properties | ||||||||||||||||||||||||||||||||||||||||||||||||||||||||||||
Name, symbol, number | rutherfordium, Rf, 104 | |||||||||||||||||||||||||||||||||||||||||||||||||||||||||||
Pronunciation | / ˌ r ʌ ð ər ˈ f ɔr d i ə m / RUDH-ər-FOR-dee-əm |
|||||||||||||||||||||||||||||||||||||||||||||||||||||||||||
Element category | transition metal | |||||||||||||||||||||||||||||||||||||||||||||||||||||||||||
Group, period, block | 4, 7, d | |||||||||||||||||||||||||||||||||||||||||||||||||||||||||||
Standard atomic weight | [267] | |||||||||||||||||||||||||||||||||||||||||||||||||||||||||||
Electron configuration | [Rn] 5f14 6d2 7s2 2, 8, 18, 32, 32, 10, 2 |
|||||||||||||||||||||||||||||||||||||||||||||||||||||||||||
History | ||||||||||||||||||||||||||||||||||||||||||||||||||||||||||||
Discovery | Joint Institute for Nuclear Research (1964) | |||||||||||||||||||||||||||||||||||||||||||||||||||||||||||
Physical properties | ||||||||||||||||||||||||||||||||||||||||||||||||||||||||||||
Phase | solid (predicted) | |||||||||||||||||||||||||||||||||||||||||||||||||||||||||||
Density (near r.t.) | 23 (estimated) g·cm−3 | |||||||||||||||||||||||||||||||||||||||||||||||||||||||||||
Melting point | 2400 K, 2100 °C, 3800 (estimated) °F | |||||||||||||||||||||||||||||||||||||||||||||||||||||||||||
Boiling point | 5800 K, 5500 °C, 9900 (estimated) °F | |||||||||||||||||||||||||||||||||||||||||||||||||||||||||||
Atomic properties | ||||||||||||||||||||||||||||||||||||||||||||||||||||||||||||
Oxidation states | 4, 3 (predicted) (only bolded oxidation states are known experimentally) |
|||||||||||||||||||||||||||||||||||||||||||||||||||||||||||
Ionization energies ( more) |
1st: 579.9 (estimated) kJ·mol−1 | |||||||||||||||||||||||||||||||||||||||||||||||||||||||||||
2nd: 1389.4 (estimated) kJ·mol−1 | ||||||||||||||||||||||||||||||||||||||||||||||||||||||||||||
3rd: 2296.4 (estimated) kJ·mol−1 | ||||||||||||||||||||||||||||||||||||||||||||||||||||||||||||
Atomic radius | 150 (estimated) pm | |||||||||||||||||||||||||||||||||||||||||||||||||||||||||||
Covalent radius | 157 (estimated) pm | |||||||||||||||||||||||||||||||||||||||||||||||||||||||||||
Miscellanea | ||||||||||||||||||||||||||||||||||||||||||||||||||||||||||||
CAS registry number | 53850-36-5 | |||||||||||||||||||||||||||||||||||||||||||||||||||||||||||
Most stable isotopes | ||||||||||||||||||||||||||||||||||||||||||||||||||||||||||||
Main article: Isotopes of rutherfordium | ||||||||||||||||||||||||||||||||||||||||||||||||||||||||||||
|
||||||||||||||||||||||||||||||||||||||||||||||||||||||||||||
Rutherfordium is a chemical element with symbol Rf and atomic number 104, named in honour of New Zealand-born British physicist Ernest Rutherford. It is a synthetic element (an element that can be created in a laboratory but is not found in nature) and radioactive; the most stable known isotope, 267Rf, has a half-life of approximately 1.3 hours.
In the periodic table of the elements, it is a d-block element and the first of the transactinide elements. It is a member of the 7th period and belongs to the group 4 elements. Chemistry experiments have confirmed that rutherfordium behaves as the heavier homologue to hafnium in group 4. The chemical properties of rutherfordium are characterized only partly. They compare well with the chemistry of the other group 4 elements, even though some calculations had indicated that the element might show significantly different properties due to relativistic effects.
In the 1960s, small amounts of rutherfordium were produced in laboratories in the former Soviet Union and in California. The priority of the discovery and therefore the naming of the element was disputed between Soviet and American scientists, and it was not until 1997 that International Union of Pure and Applied Chemistry (IUPAC) established rutherfordium as the official name for the element.
History
Discovery
Rutherfordium was reportedly first detected in 1964 at the Joint Institute of Nuclear Research at Dubna (then in the Soviet Union). Researchers there bombarded a plutonium-242 target with neon-22 ions and separated the reaction products by gradient thermochromatography after conversion to chlorides by interaction with ZrCl4. The team identified spontaneous fission activity contained within a volatile chloride portraying eka-hafnium properties. Although a half-life was not accurately determined, later calculations indicated that the product was most likely rutherfordium-259 (abbreviated as 259Rf in standard notation):
- 242
94Pu + 22
10Ne → 264−x
104Rf → 264−x
104RfCl4
In 1969, researchers at the University of California, Berkeley conclusively synthesized the element by bombarding a californium-249 target with carbon-12 ions and measured the alpha decay of 257Rf, correlated with the daughter decay of nobelium-253:
- 249
98Cf + 12
6C → 257
104Rf + 4 n
The American synthesis was independently confirmed in 1973 and secured the identification of rutherfordium as the parent by the observation of K-alpha X-rays in the elemental signature of the 257Rf decay product, nobelium-253.
Naming controversy
The Russian scientists proposed the name kurchatovium and the American scientists suggested the name rutherfordium for the new element. In 1992, the IUPAC/ IUPAP Transfermium Working Group (TWG) assessed the claims of discovery and concluded that both teams provided contemporaneous evidence to the synthesis of element 104 and that credit should be shared between the two groups.
The American group wrote a scathing response to the findings of the TWG, stating that they had given too much emphasis on the results from the Dubna group. In particular they pointed out that the Russian group had altered the details of their claims several times over a period of 20 years, a fact that the Russian team does not deny. They also stressed that the TWG had given too much credence to the chemistry experiments performed by the Russians and accused the TWG of not having appropriately qualified personnel on the committee. The TWG responded by saying that this was not the case and having assessed each point raised by the American group said that they found no reason to alter their conclusion regarding priority of discovery. The IUPAC finally used the name suggested by the American team (rutherfordium) which may in some way reflect a change of opinion.
As a consequence of the initial competing claims of discovery, an element naming controversy arose. Since the Soviets claimed to have first detected the new element they suggested the name kurchatovium, Ku, in honour of Igor Kurchatov (1903–1960), former head of Soviet nuclear research. This name had been used in books of the Soviet Bloc as the official name of the element. The Americans, however, proposed rutherfordium (Rf) for the new element to honour Ernest Rutherford, who is known as the "father" of nuclear physics. The International Union of Pure and Applied Chemistry ( IUPAC) adopted unnilquadium, Unq, as a temporary, systematic element name, derived from the Latin names for digits 1, 0, and 4. In 1994, IUPAC suggested the name dubnium to be used since rutherfordium was suggested for element 106 and IUPAC felt that the Dubna team should be rightly recognized for their contributions. However, there was still a dispute over the names of elements 104–107. In 1997 the teams involved resolved the dispute and adopted the current name rutherfordium. The name dubnium was given to element 105 at the same time.
Nucleosynthesis
Super-heavy elements such as rutherfordium are produced by bombarding lighter elements in particle accelerators that induces fusion reactions. Whereas most of the isotopes of rutherfordium can be synthesized directly this way, some heavier ones have only been observed as decay products of elements with higher atomic numbers.
Depending on the energies involved, the former are separated into "hot" and "cold". In hot fusion reactions, very light, high-energy projectiles are accelerated toward very heavy targets (actinides), giving rise to compound nuclei at high excitation energy (~40–50 MeV) that may either fission or evaporate several (3 to 5) neutrons. In cold fusion reactions, the produced fused nuclei have a relatively low excitation energy (~10–20 MeV), which decreases the probability that these products will undergo fission reactions. As the fused nuclei cool to the ground state, they require emission of only one or two neutrons, and thus, allows for the generation of more neutron-rich products. The latter is a distinct concept from that of where nuclear fusion claimed to be achieved at room temperature conditions (see cold fusion).
Hot fusion studies
The synthesis of rutherfordium was first attempted in 1964 by the team at Dubna using the hot fusion reaction of neon-22 projectiles with plutonium-242 targets:
- 242
94Pu + 22
10Ne → 264-x
104Rf + 3 or 5 n.
The first study produced evidence for a spontaneous fission with a 0.3 second half-life and another one at 8 seconds. While the former observation was eventually retracted, the latter eventually became associated with the 259Rf isotope. In 1966, the Soviet team repeated the experiment using a chemical study of volatile chloride products. They identified a volatile chloride with eka-hafnium properties that decayed fast through spontaneous fission. This gave strong evidence for the formation of RfCl4, and although a half-life was not accurately measured, later evidence suggested that the product was most likely 259Rf. The team repeated the experiment several times over the next few years, and in 1971, they revised the spontaneous fission half time for the isotope at 4.5 seconds.
In 1969, researchers at the University of California led by Albert Ghiorso, tried to confirm the original results reported at Dubna. In a reaction of curium-248 with oxygen-16, they were unable to confirm the result of the Soviet team, but managed to observe the spontaneous fission of 260Rf with a very short half-life of 10–30 ms:
- 248
96Cm + 16
8O → 260
104Rf + 4 n.
In 1970, the American team also studied the same reaction with oxygen-18 and identified 261Rf with a half-life of 65 seconds (later refined to 75 seconds). Later experiments at the Lawrence Berkeley National Laboratory in California also revealed the formation of a short-lived isomer of 262Rf (which undergoes spontaneous fission with a half-life of 47 ms), and spontaneous fission activities with long lifetimes tentatively assigned to 263Rf.
The reaction of californium-249 with carbon-13 was also investigated by the Ghiorso team, which indicated the formation of the short-lived 258Rf (which undergoes spontaneous fission in 11 ms):
- 249
98Cf + 13
6C → 258
104Rf + 4 n.
In trying to confirm these results by using carbon-12 instead, they also observed the first alpha decays from 257Rf.
The reaction of berkelium-249 with nitrogen-14 was first studied in Dubna in 1977, and in 1985, researchers there confirmed the formation of the 260Rf isotope which quickly undergoes spontaneous fission in 28 ms:
- 249
97Bk + 14
7N → 260
104Rf + 3 n.
In 1996 the isotope 262Rf was observed in LBNL from the fusion of plutonium-244 with neon-22:
- 244
94Pu + 22
10Ne → 266-x
104Rf + 4 or 5 n.
The team determined a half-life of 2.1 seconds, in contrast to earlier reports of 47 ms and suggested that the two half-lives might be due to different isomeric states of 262Rf. Studies on the same reaction by a team at Dubna, lead to the observation in 2000 of alpha decays from 261Rf and spontaneous fissions of 261mRf.
The hot fusion reaction using a uranium target was first reported at Dubna in 2000:
- 238
92U + 26
12Mg → 264-x
104Rf + x n (x = 3, 4, 5, 6).
They observed decays from 260Rf and 259Rf, and later for 259Rf. In 2006, as part of their program on the study of uranium targets in hot fusion reactions, the team at LBNL also observed 261Rf.
Cold fusion studies
The first cold fusion experiments involving element 104 were done in 1974 at Dubna, by using light titanium-50 nuclei aimed at lead-208 isotope targets:
- 208
82Pb + 50
22Ti → 258-x
104Rf + x n (x = 1, 2, or 3).
The measurement of a spontaneous fission activity was assigned to 256Rf, while later studies done at the Gesellschaft für Schwerionenforschung Institute (GSI), also measured decay properties for the isotopes 257Rf, and 255Rf.
In 1974 researchers at Dubna investigated the reaction of lead-207 with titanium-50 to produce the isotope 255Rf. In a 1994 study at GSI using the lead-206 isotope, 255Rf as well as 254Rf were detected. 253Rf was similarly detected that year when lead-204 was used instead.
Decay studies
Most isotopes with an atomic mass below 262 have also observed as decay products of elements with a higher atomic number, allowing for refinement of their previously measured properties. Heavier isotopes of rutherfordium have only been observed as decay products. For example, a few alpha decay events terminating in 267Rf were observed in the decay chain of darmstadtium-279 since 2004:
- 279
110Ds → 275
108Hs + α → 271
106Sg + α → 267
104Rf + α.
This further underwent spontaneous fission with a half-time of about 1.3 h.
Investigations on the synthesis of the dubnium-263 isotope in 1999 at the University of Bern revealed events consistent with electron capture to form 263Rf. A rutherfordium fraction was separated, and several spontaneous fission events with long lifetimes of about 15 minutes were observed, as well as and alpha decays with lifetimes of about 10 minutes. Reports on the decay chain of flerovium-285 in 2010 showed five sequential alpha decays that terminate in 265Rf, which further undergoes spontaneous fission with a life-time of 152 seconds.
Some experimental evidence was obtained in 2004 for an even heavier isotope, 268Rf, in the decay chain of an isotope of ununpentium:
- 288
115Uup → 284
113Uut + α → 280
111Rg + α → 276
109Mt + α → 272
107Bh + α → 268
105Db + α ? → 268
104Rf + ν
e.
However, the last step in this chain was uncertain. After observing the five alpha decay events that generate dubnium-268, spontaneous fission events were observed with a long half-time. It is unclear whether these events were due to direct spontaneous fission of 268Db, or 268Db produced electron capture events with long half-times to generate 268Rf. If the latter is produced and decays with a short life-time, the two possibilities cannot be distinguished. Given that the electron capture of 268Db cannot be detected, these spontaneous fission events may be due to 268Rf, in which case the half-life of this isotope cannot be extracted.
According to a 2007 report on the synthesis of ununtrium, the isotope 282113 was observed to undergo a similar decay to form 266Db, which undergoes spontaneous fission with a half-life of 22 minutes. Given that the electron capture of 266Db cannot be detected, these spontaneous fission events may be due to 266Rf, in which case the half-life of this isotope cannot be extracted.
Isotopes
Isotope |
Half-life |
Decay mode |
Discovery year |
Reaction |
---|---|---|---|---|
253Rf | 48 μs | α, SF | 1994 | 204Pb(50Ti,n) |
254Rf | 23 μs | SF | 1994 | 206Pb(50Ti,2n) |
255Rf | 2.3 s | ε?, α, SF | 1974 | 207Pb(50Ti,2n) |
256Rf | 6.4 ms | α, SF | 1974 | 208Pb(50Ti,2n) |
257Rf | 4.7 s | ε, α, SF | 1969 | 249Cf(12C,4n) |
257mRf | 4.1 s | ε, α, SF | 1969 | 249Cf(12C,4n) |
258Rf | 14.7 ms | α, SF | 1969 | 249Cf(13C,4n) |
259Rf | 3.2 s | α, SF | 1969 | 249Cf(13C,3n) |
259mRf | 2.5 s | ε | 1969 | 249Cf(13C,3n) |
260Rf | 21 ms | α, SF | 1969 | 248Cm(16O,4n) |
261Rf | 78 s | α, SF | 1970 | 248Cm(18O,5n) |
261mRf | 4 s | ε, α, SF | 2001 | 244Pu(22Ne,5n) |
262Rf | 2.3 s | α, SF | 1996 | 244Pu(22Ne,4n) |
263Rf | 15 min | α, SF | 1999 | 263Db(e−, ν e) |
263mRf ? | 8 s | α, SF | 1999 | 263Db(e−, ν e) |
264Rf | 1? h | α ? | unknown | — |
265Rf | 2.5 min | SF | 2010 | 269Sg(—,α) |
266Rf | 10 h ? | α, SF ? | 2007? | 266Db(e−, ν e)? |
267Rf | 1.3 h | SF | 2004 | 271Sg(—,α) |
268Rf | 6 h ? | α, SF ? | 2004? | 268Db(e−, ν e)? |
Rutherfordium has no stable or naturally-occurring isotopes. Several radioactive isotopes have been synthesized in the laboratory, either by fusing two atoms or by observing the decay of heavier elements. Fifteen different isotopes have been reported with atomic masses from 253 to 268 (with the exception of 264). Most of these decay predominantly through spontaneous fission pathways.
Life-times
The lighter isotopes usually have shorter half-lives; half-lives of under 50 μs for 253Rf and 254Rf were observed. 256Rf, 258Rf, 260Rf are more stable at around 10 ms, 255Rf, 257Rf, 259Rf, and 262Rf live between 1 and 5 seconds, and 261Rf, 265Rf, and 263Rf are more stable, at around 1, 1.5, and 10 min respectively. The heaviest isotopes are the most stable, with 267Rf having a measured half-life of about 1.3 h.
The lightest isotopes were synthesized by direct fusion between two lighter nuclei and as decay products. The heaviest isotope produced by direct fusion is 262Rf; heavier isotopes have only been observed as decay products of elements with larger atomic numbers, of which only 267Rf has been confirmed. Isotopes 266Rf and 268Rf may have also been observed as decay products and are thought to have long half-lives of 10 h and 6 h, respectively—but these have been measured indirectly, through systematic studies. While the isotope 264Rf has yet to be observed, it is predicted to have a long half-life of 1 h. Before its discovery, 265Rf was predicted to have an even longer half-life of 13 h, but it has a half-life of only 2.5 min.
In 1999, American scientists at the University of California, Berkeley, announced that they had succeeded in synthesizing three atoms of 293118. These parent nuclei were reported to have successively emitted seven alpha particles to form 265Rf nuclei, but their claim was retracted in 2001.
Nuclear isomerism
Several early studies on the synthesis of 263Rf have indicated that this nuclide decays primarily by spontaneous fission with a half-life of 10–20 minutes. More recently, a study of hassium isotopes allowed the synthesis of atoms of 263Rf decaying with a shorter half-life of 8 seconds. These two different decay modes must be associated with two isomeric states, but specific assignments are difficult due to the low number of observed events.
During research on the synthesis of rutherfordium isotopes utilizing the 244Pu(22Ne,5n)261Rf reaction, the product was found to undergo exclusive 8.28 MeV alpha decay with a half-life of 78 seconds. Later studies at GSI on the synthesis of copernicium and hassium isotopes produced conflicting data, as 261Rf produced in the decay chain was found to undergo 8.52 MeV alpha decay with a half-life of 4 seconds. Later results indicated a predominant fission branch. These contradictions led to some doubt on the discovery of copernicium. The first isomer is currently denoted 261aRf (or simply 261Rf) whilst the second is denoted 261bRf (or 261mRf). However, it is thought that the first nucleus belongs to a high-spin ground state and the latter to a low-spin metastable state. The discovery and confirmation of 261bRf provided proof for the discovery of copernicium in 1996.
A detailed spectroscopic study of the production of 257Rf nuclei using the reaction 208Pb(50Ti,n)257Rf allowed the identification of an isomeric level in 257Rf. The work confirmed that 257gRf has a complex spectrum with 15 alpha lines. A level structure diagram was calculated for both isomers. Similar isomers were reported for 256Rf also.
Predicted properties
Chemical
Rutherfordium is the first transactinide element and the second member of the 6d series of transition metals. Calculations on its ionization potentials, atomic radius, as well as radii, orbital energies, and ground levels of its ionized states are similar to that of hafnium and very different from that of lead. Therefore it was concluded that rutherfordium's basic properties will resemble those of other group 4 elements, below titanium, zirconium, and hafnium. Some of its properties were determined by gas-phase experiments and aqueous chemistry. The oxidation state +4 is the only stable state for the latter two elements and therefore rutherfordium should also exhibit a stable +4 state. In addition, rutherfordium is also expected to be able to form a less stable +3 state.
The chemical properties of rutherfordium were based on calculation which indicated that the relativistic effects on the electron shell might be strong enough that the p orbitals have a lower energy level than the d orbitals, giving it an valence electron configuration of 6d1 7s2 7p1 or even 7s2 7p2, therefore making the element behave more like lead than hafnium. With better calculation methods and studies of the chemical properties of rutherfordium compounds it could be shown that rutherfordium behaves according to the rest of the group 4 elements.
In an analogous manner to zirconium and hafnium, rutherfordium is projected to form a very stable, high melting point oxide, RfO2. It reacts with halogens to form tetrahalides, RfX4, which hydrolyze on contact with water to form oxyhalides RfOX2. The tetrahalides are volatile solids existing as monomeric tetrahedral molecules in the vapor phase.
In the aqueous phase, the Rf4+ ion hydrolyzes less than titanium(IV) and to a similar extent as zirconium and hafnium, thus resulting in the RfO2+ ion. Treatment of the halides with halide ions promotes the formation of complex ions. The use of chloride and bromide ions produces the hexahalide complexes RfCl2−
6 and RfBr2−
6. For the fluoride complexes, zirconium and hafnium tend to form hepta- and octa- complexes. Thus, for the larger rutherfordium ion, the complexes RfF2−
6, RfF2−
7 and RfF4−
8 are possible.
Physical and atomic
Rutherfordium is expected to be a solid under normal conditions. It should be a very heavy metal with a density of around 23 g/cm3; in comparison, the densest known element that has had its density measured, osmium, has a density of 22.61 g/cm3. This results from rutherfordium's high atomic weight, the lanthanide and actinide contractions, and relativistic effects, although production of enough rutherfordium to measure this quantity would be impractical, and the sample would quickly decay. The atomic radius for rutherfordium is expected to be around 150 pm. Due to the relativistic stabilization of the 7s orbital and destabilization of the 6d orbital, the Rf+ and Rf2+ ions are predicted to give up 6d electrons instead of 7s electrons, which is the opposite of the behaviour of its lighter homologues.
Experimental chemistry
Formula | Names |
---|---|
RfCl4 | rutherfordium tetrachloride, rutherfordium(IV) chloride |
RfBr4 | rutherfordium tetrabromide, rutherfordium(IV) bromide |
RfOCl2 | rutherfordium oxychloride, rutherfordyl(IV) chloride, rutherfordium(IV) dichloride oxide |
[RfCl6]2− | hexachlororutherfordate(IV) |
[RfF6]2− | hexafluororutherfordate(IV) |
K2[RfCl6] | potassium hexachlororutherfordate(IV) |
Gas phase
Early work on the study of the chemistry of rutherfordium focused on gas thermochromatography and measurement of relative deposition temperature adsorption curves. The initial work was carried out at Dubna in an attempt to reaffirm their discovery of the element. Recent work is more reliable regarding the identification of the parent rutherfordium radioisotopes. The isotope 261mRf has been used for these studies. The experiments relied on the expectation that rutherfordium would begin the new 6d series of elements and should therefore form a volatile tetrachloride due to the tetrahedral nature of the molecule. Rutherfordium(IV) chloride is more volatile than its lighter homologue hafnium(IV) chloride (HfCl4) because its bonds are more covalent.
A series of experiments confirmed that rutherfordium behaves as a typical member of group 4, forming a tetravalent chloride (RfCl4) and bromide (RfBr4) as well as an oxychloride (RfOCl2). A decreased volatility was observed for RfCl4 when potassium chloride is provided as the solid phase instead of gas, highly indicative of the formation of nonvolatile K2RfCl6 mixed salt.
Aqueous phase
Rutherfordium is expected to have the electron configuration [Rn]5f14 6d2 7s2 and therefore behave as the heavier homologue of hafnium in group 4 of the periodic table. It should therefore readily form a hydrated Rf4+ ion in strong acid solution and should readily form complexes in hydrochloric acid, hydrobromic or hydrofluoric acid solutions.
The most conclusive aqueous chemistry studies of rutherfordium have been performed by the Japanese team at Japan Atomic Energy Research Institute using the radioisotope 261mRf. Extraction experiments from hydrochloric acid solutions using isotopes of rutherfordium, hafnium, zirconium, and thorium have proved a non-actinide behaviour for rutherfordium. A comparison with its lighter homologues placed rutherfordium firmly in group 4 and indicated the formation of a hexachlororutherfordate complex in chloride solutions, in a manner similar to hafnium and zirconium.
- 261mRf4+ + 6 Cl− → [261mRfCl6]2−
Very similar results were observed in hydrofluoric acid solutions. Differences in the extraction curves were interpreted as a weaker affinity for fluoride ion and the formation of the hexafluororutherfordate ion, whereas hafnium and zirconium ions complex seven or eight fluoride ions at the concentrations used:
- 261mRf4+ + 6 F− → [261mRfF6]2−