Proteomics is the large-scale study of proteins, particularly their structures and functions. The proteome is the entire complement of proteins, including the modifications made to a particular set of proteins, produced by an organism or system. This will vary with time and distinct requirements, or stresses, that a cell or organism undergoes.
While proteomics generally refers to the large-scale experimental analysis of proteins, it is often specifically used for protein purification and mass spectrometry. After genomics and transcriptomics, proteomics is considered the next step in the study of biological systems. It is much more complicated than genomics mostly because while an organism's genome is more or less constant, the proteome differs from cell to cell and from time to time. This is because distinct genes are expressed in distinct cell types. This means that even the basic set of proteins which are produced in a cell needs to be determined. In the past, this was done by mRNA analysis, but this was found not to correlate with protein content. It is now known that mRNA is not always translated into protein. The amount of protein produced for a given amount of mRNA depends on the gene it is transcribed from and the current physiological state of the cell.
Proteomics confirms the presence of the protein and provides a direct measure of the quantity present. Not only does the translation from mRNA cause differences, but many proteins are also subjected to a wide variety of chemical modifications after translation which are critical to the protein's function such as phosphorylation, ubiquitination, methylation, acetylation, glycosylation, oxidation, and nitrosylation. Some proteins undergo ALL of these modifications, often in time-dependent combinations, aptly illustrating the potential complexity one has to deal with when studying protein structure and function.
Proteomics typically gives us a better understanding of an organism than genomics. First, the level of transcription of a gene gives only a rough estimate of its level of expression into a protein. An mRNA produced in abundance may be degraded rapidly or translated inefficiently, resulting in a small amount of protein. Second, as mentioned above many proteins experience post-translational modifications that profoundly affect their activities. For example, some proteins are not active until they become phosphorylated. Third, many transcripts give rise to more than one protein through alternative splicing or alternative post-translational modifications. Fourth, many proteins form complexes with other proteins or RNA molecules. They only function in the presence of these other molecules. Finally, protein degradation rate plays an important role in protein content.
One way in which a particular protein can be studied is to develop an antibody which is specific to that modification. For example, there are antibodies that only recognize certain proteins when they are tyrosine-phosphorylated, known as phospho-specific antibodies. There are also antibodies specific to other modifications. These can be used to determine the set of proteins that have undergone the modification of interest. For more quantitative determinations of protein amounts, techniques such as ELISAs can be used.
Most proteins function in collaboration with other proteins. One goal of proteomics is to identify which proteins interact. This is especially useful in determining potential partners in cell signaling cascades. Several methods are available to probe protein–protein interactions. The traditional method is yeast two-hybrid analysis. New methods include protein microarrays, immunoaffinity, and chromatography followed by mass spectrometry , dual polarisation interferometry, Microscale Thermophoresis, and experimental methods such as phage display and computational methods.
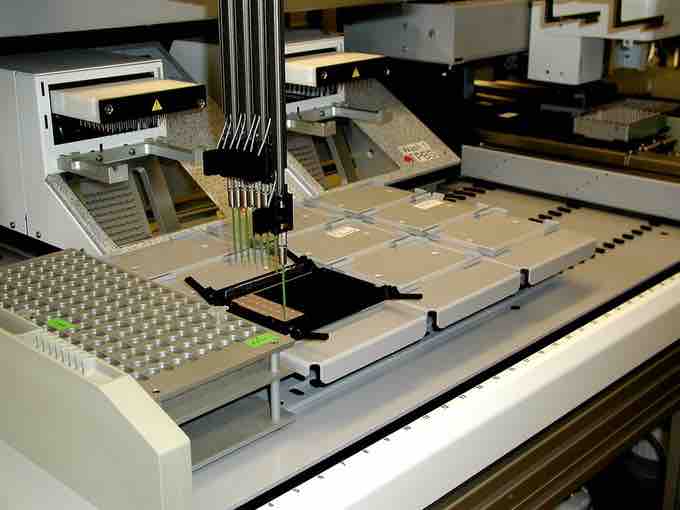
Robotic preparation of MALDI mass spectrometry samples
Matrix-assisted laser desorption/ionization (MALDI) is a soft ionization technique used in mass spectrometry. It allows for the analysis of biomolecules and large organic molecules which tend to be fragile and fragment when ionized by more conventional ionization methods.
One of the most promising developments to come from the study of human genes and proteins has been the identification of potential new drugs for the treatment of disease. This relies on genome and proteome information to identify proteins associated with a disease, which computer software can then use as targets for new drugs. For example, if a certain protein is implicated in a disease, its 3-D structure provides the information to design drugs to interfere with the action of the protein. A molecule that fits the active site of an enzyme, but cannot be released by the enzyme, will inactivate the enzyme. Understanding the proteome, the structure and function of each protein and the complexities of protein–protein interactions will be critical for developing the most effective diagnostic techniques and disease treatments in the future. Moreover, an interesting use of proteomics is using specific protein biomarkers to diagnose disease. A number of techniques allow testing for proteins produced during a particular disease, which helps to diagnose the disease quickly.