Proton Gradients in Reductive Metabolism
Biological energy is frequently stored and released by means of redox reactions, or the transfer of electrons. Reduction occurs when an oxidant gains an electron. Photosynthesis involves the reduction of carbon dioxide into sugars and the oxidation of water into molecular oxygen. The reverse reaction, respiration, oxidizes sugars (loses an electron) to produce carbon dioxide and water. As intermediate steps, the reduced carbon compounds are used to reduce nicotinamide adenine dinucleotide (NAD+), which then contributes to the creation of a proton gradient. This then drives the synthesis of adenosine triphosphate (ATP) and is maintained by the reduction of oxygen, or alternative receptors for anaerobic respiration. In animal cells, the mitochondria performs similar functions.
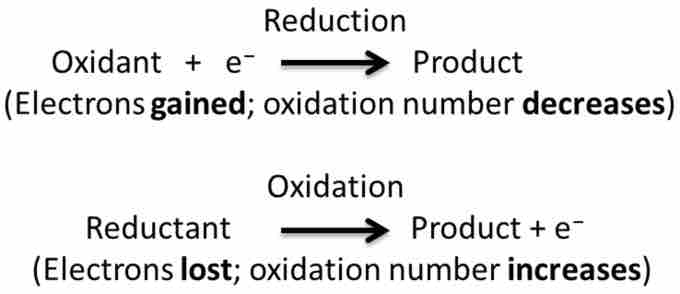
The Basics of Redox
In every redox reaction you have two halves: reduction and oxidation.
An electrochemical gradient represents one of the many interchangeable forms of potential energy through which energy may be conserved. In biological processes, the direction an ion moves by diffusion or active transport across a membrane is determined by the electrochemical gradient. In the mitochondria and chloroplasts, proton gradients are used to generate a chemiosmotic potential that is also known as a proton motive force. This potential energy is used for the synthesis of ATP by phosphorylation. An electrochemical gradient has two components. First, the electrical component is caused by a charge difference across the lipid membrane. Second, a chemical component is caused by a differential concentration of ions across the membrane. The combination of these two factors determines the thermodynamically favorable direction for an ion's movement across a membrane. The electrochemical potential difference between the two sides of the membrane in mitochondria, chloroplasts, bacteria, and other membranous compartments that engage in active transport involving proton pumps, is at times called a chemiosmotic potential or proton motive force.
In respiring bacteria under physiological conditions, ATP synthase, in general, runs in the opposite direction, creating ATP while using the proton motive force created by the electron transport chain as a source of energy. The overall process of creating energy in this fashion is termed oxidative phosphorylation. The same process takes place in the mitochondria, where ATP synthase is located in the inner mitochondrial membrane, so that F1 part sticks into the mitochondrial matrix where ATP synthesis takes place.
Cellular respiration (both aerobic and anaerobic) utilizes highly reduced species such as NADH and FADH2 to establish an electrochemical gradient (often a proton gradient) across a membrane, resulting in an electrical potential or ion concentration difference across the membrane. The reduced species are oxidized by a series of respiratory integral membrane proteins with sequentially increasing reduction potentials, the final electron acceptor being oxygen (in aerobic respiration) or another species (in anaerobic respiration). The membrane in question is the inner mitochondrial membrane in eukaryotes and the cell membrane in prokaryotes. A proton motive force or pmf drives protons down the gradient (across the membrane) through the proton channel of ATP synthase. The resulting current drives ATP synthesis from ADP and inorganic phosphate.
Proton reduction is important for setting up electrochemical gradients for anaerobic respiration. For example, in denitrification, protons are transported across the membrane by the initial NADH reductase, quinones, and nitrous oxide reductase to produce the electrochemical gradient critical for respiration. In organisms that use hydrogen as an energy source, hydrogen is oxidized by a membrane-bound hydrogenase causing proton pumping via electron transfer to various quinones and cytochromes. Sulfur oxidation is a two step process that occurs because energetically sulfide is a better electron donor than inorganic sulfur or thiosulfate, allowing for a greater number of protons to be translocated across the membrane.
In contrast, fermentation does not utilize an electrochemical gradient. Instead, it only uses substrate-level phosphorylation to produce ATP. The electron acceptor NAD+ is regenerated from NADH formed in oxidative steps of the fermentation pathway by the reduction of oxidized compounds. These oxidized compounds are often formed during the fermentation pathway itself, but may also be external. For example, in homofermentative lactic acid bacteria, NADH formed during the oxidation of glyceraldehyde-3-phosphate is oxidized back to NAD+ by the reduction of pyruvate to lactic acid at a later stage in the pathway. In yeast, acetaldehyde is reduced to ethanol.