The d-Block of the Periodic Table
The transition metals are also known as thetransition elements or the d-block elements. As the name implies, the chemistry of this group is determined by the extent to which the d-electron suborbital levels are filled. Chemical similarities and periodicities can be easily seen horizontally across the d-block of the periodic table.
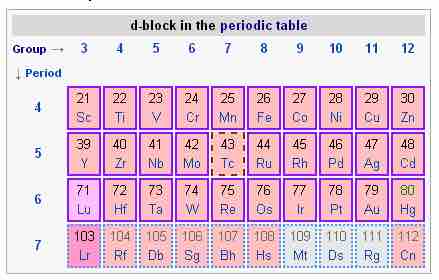
The d-block of the periodic table
The d-block is composed of groups 3 - 12 and periods 4 - 7.
The chemistry is far from simple, however, and there are many exceptions to the orderly filling of the electron shell. The Aufbau principle provides an methodical framework for predicting the order in which most atoms will populate their electron shells.
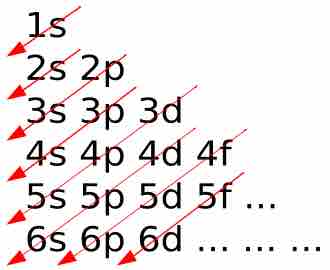
The Aufbau principle
This illustrates the order in which most atoms populate their electron shells.
Chemical properties in the periodic table are organized vertically, by group, for similar chemical and physical properties. For example, the metals in group 11 have similar characteristics of electrical conductivity, luster, crystal structure, ductility, and tensile strength. Moving horizontally across the periodic table trends in properties such as atomic radius, electronegativity, and electron affinity are observed.
Characteristic Properties of Transition Metals
Transition metals can be said to possess the following characteristics generally not found in the main grouping of the periodic table. They can be mostly attributed to incomplete filling of the electron d-levels:
- The formation of compounds whose color is due to d–d electronic transitions.
- The formation of compounds in many oxidation states due to the relatively low reactivity of unpaired d electrons.
- The formation of many paramagnetic compounds due to the presence of unpaired d electrons. A few compounds of main group elements are also paramagnetic (e.g., nitric oxide, oxygen).
Ligand-to-Metal Charge-Transfer (LMCT) Transition
Color in transition-series metal compounds is generally due to the electronic transitions of two principal types of charge transfer transitions. An electron may jump from a predominantly ligand orbital to a predominantly metal orbital, giving rise to a ligand-to-metal charge transfer (LMCT) transition. These can most easily occur when the metal is in a high oxidation state. For example, the color of chromate, dichromate, and permanganate ions is due to LMCT transitions. Another example is that mercuric iodide (HgI2) is red because of a LMCT transition.
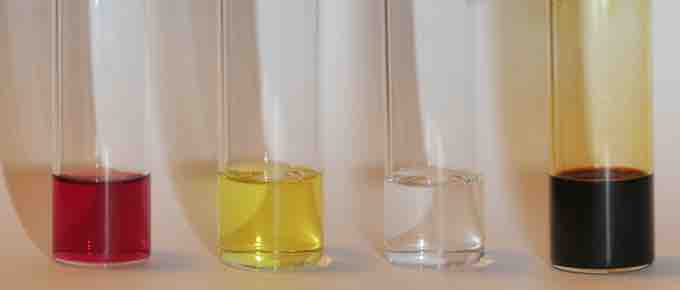
Charge-transfer complexes
I2•PPh3 charge-transfer complexes in CH2Cl2. From left to right: (1) I2 dissolved in dichloromethane—no CT complex. (2) A few seconds after excess PPh3 was added—CT complex is forming. (3) One minute later after excess PPh3 was added—the CT complex [Ph3PI]+I-has been formed. (4) Immediately after excess I2 was added, which contains [Ph3PI]+[I3]-.
A metal-to-ligand charge transfer (MLCT) transition will be most likely when the metal is in a low oxidation state and the ligand is an easily reduced d-d transition. An electron jumps from one d-orbital to another. In complexes of the transition metals, the d orbitals do not all have the same energy.
Paramagnetic and Diamagnetic Compounds
Transition metal compounds are paramagnetic when they have one or more unpaired d electrons. Some compounds are diamagnetic. These include octahedral, low-spin, d6 and square-planar d8complexes. In these cases, crystal field splitting is such that all the electrons are paired up. Ferromagnetism occurs when individual atoms are paramagnetic and the spin vectors are aligned parallel to each other in a crystalline material. Metallic iron and the alloy alnico are examples of ferromagnetic materials involving transition metals. Anti-ferromagnetism is another example of a magnetic property arising from a particular alignment of individual spins in the solid state.
The transition metals and their compounds are known for their homogeneous and heterogeneous catalytic activity. This activity is attributed to their ability to adopt multiple oxidation states and to form complexes.