Henry's Law
Henry's law states that at a constant temperature, the amount of a gas that dissolves in a liquid is directly proportional to the partial pressure of that gas in equilibrium with that liquid. It was formulated by William Henry in 1803.
The practical description for the law is that the solubility (i.e., equilibrium) of a gas in a liquid is directly proportional to the partial pressure of that gas. In addition, the partial pressure is able to predict the tendency to dissolve simply because the gasses with higher partial pressures have more molecules and will bounce into the solution they can dissolve into more often than gasses with lower partial pressures.
Henry's law also applies to the solubility of other substances that aren't gaseous, such as the equilibrium of organic pollutants in water being based on the relative concentration of that pollutant in the media its suspended in.
Henry's law can be put into mathematical terms (at constant temperature):
Where p is the partial pressure of the solute in the gas above the solution, c is the concentration of the solute, the solubility of the substance is k, and the Henry's law constant (H), which depends on the solute, the solvent, and the temperature.
The solubility captures the tendency of a substance to go towards equilibrium in a solution, which explains why gasses that have the same partial pressure may have different tendencies to dissolve.
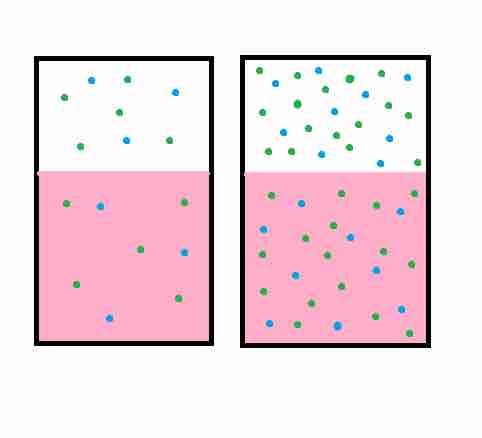
Henry's law
Henry's law states that when a gas is in contact with the surface of a liquid, the amount of the gas which will go into solution is proportional to the partial pressure of that gas.
Henry's Law in Respiration
The main application of Henry's law in respiratory physiology is to predict how gasses will dissolve in the alveoli and bloodstream during gas exchange. The amount of oxygen that dissolves into the bloodstream is directly proportional to the partial pressure of oxygen in alveolar air.
The partial pressure of oxygen is greater in alveolar air than in deoxygenated blood, so oxygen has a high tendency to dissolve into deoxygenated blood. Conversely the opposite is true for carbon dioxide, which has a greater partial pressure in deoxygenated blood than in the alveolar air, so it will diffuse out of the solution and back into gaseous form.
Recall that the difference in partial pressures between the bloodstream and alveoli (the partial pressure gradient) are much smaller for carbon dioxide compared to oxygen. Carbon dioxide has much higher solubility in the plasma of blood than oxygen (roughly 22 times greater), so more carbon dioxide molecules are able to diffuse across the small pressure gradient of the capillary and alveoli.
Oxygen has a larger partial pressure gradient to diffuse into the bloodstream, so it's lower solubility in blood doesn't hinder it during gas exchange. Therefore, based on the properties of Henry's law, both the partial pressure and solubility of the oxygen and carbon dioxide determine how they will behave during gas exchange.