Nerve Conduction and Electrocardiograms
Nerve Conduction
Electric currents in the complex system of nerves in our body allow us to sense the world, control parts of our body, and think. There are three major functions of nerves. First, nerves carry messages from our sensory organs to the central nervous system, consisting of the brain and spinal cord. Second, nerves carry messages from the central nervous system to muscles and other organs. Third, nerves transmit and process signals within the central nervous system.
Nerve conduction is a general term for electrical signals carried by nerve cells. A voltage is created across the cell membrane of a neuron in its resting state. This membrane separates electrically neutral fluids having differing concentrations of ions, the most important varieties being Na+, K+, and Cl− (these are sodium, potassium, and chlorine ions). Free ions will diffuse from a region of high concentration to one of low concentration. The cell membrane is semipermeable, meaning that some ions may cross it while others cannot. In its resting state, the cell membrane is permeable to K+ and Cl−, and impermeable to Na+. Diffusion of K+ and Cl− thus creates the layers of positive and negative charge on the outside and inside of the membrane, and the Coulomb force prevents the ions from diffusing across in their entirety .
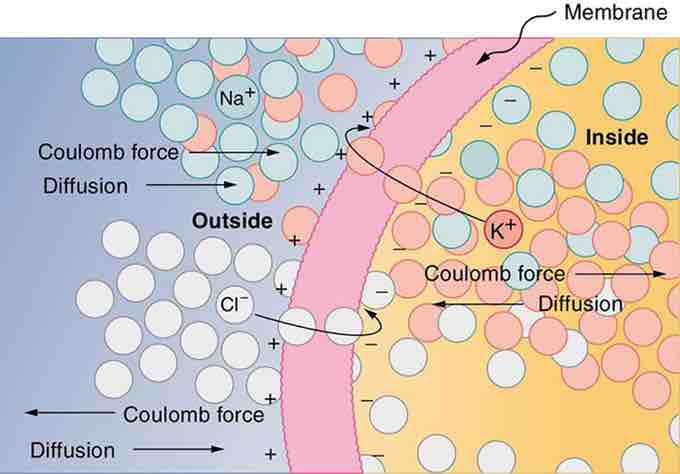
Creating a Voltage Across a Cell Membrane
The semipermeable membrane of a cell has different concentrations of ions inside and out. Diffusion moves the K+ and Cl− ions in the direction shown, until the Coulomb force halts further transfer. This results in a layer of positive charge on the outside, a layer of negative charge on the inside, and thus a voltage across the cell membrane. The membrane is normally impermeable to Na+.
Once the charge layer has built up, the repulsion of like charges prevents more from moving across, and the attraction of unlike charges prevents more from leaving either side. The result is two layers of charge right on the membrane, with diffusion being balanced by the Coulomb force. A tiny fraction of the charges move across and the fluids remain neutral, while a separation of charge and a voltage have been created across the membrane. Electric currents along the cell membrane are created by any stimulus that changes the membrane's permeability. The membrane thus temporarily becomes permeable to Na+, which then rushes in, driven both by diffusion and the Coulomb force. This inrush of Na+ first neutralizes the inside membrane (called depolarization), and then makes it slightly positive. The depolarization causes the membrane to again become impermeable to Na+, and the movement of K+ quickly returns the cell to its resting potential, referred to as repolarization. This sequence of events results in a voltage pulse, called the action potential and is shown in .
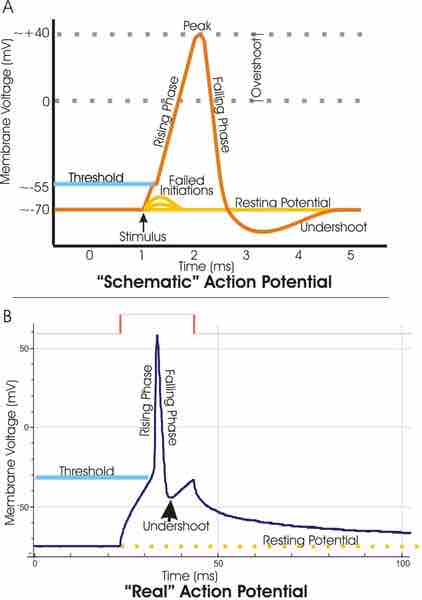
Voltage channels are critical in the generation of an action potential
Top: view of an idealized action potential shows its various phases as the action potential passes a point on a cell membrane. Bottom: Recordings of action potentials are often distorted compared to the schematic view because of variations in electrophysiological techniques used to make the recording.
Only small fractions of the ions move, so that the cell can fire many hundreds of times without depleting the excess concentrations of Na+ and K+. This is an example of active transport, wherein cell energy is used to move ions across membranes against diffusion gradients and the Coulomb force. The action potential is a voltage pulse at one location on a cell membrane.
How does it get transmitted along the cell membrane as a nerve impulse? The changing voltage and electric fields affect the permeability of the adjacent cell membrane, so that the same process takes place there. The adjacent membrane depolarizes, affecting the membrane farther down, and so on. Thus the action potential stimulated at one location triggers a nerve impulse that moves slowly (about 1 m/s) along the cell membrane.
Electrocardiograms
Just as nerve impulses are transmitted by depolarization and repolarization of an adjacent membrane, the depolarization that causes muscle contraction can also stimulate adjacent muscle cells to depolarize (fire) and contract. Thus, a depolarization wave can be sent across the heart, coordinating its rhythmic contractions and enabling it to perform its vital function of propelling blood through the circulatory system. An electrocardiogram (ECG) is a record of the voltages created by the wave of depolarization (and subsequent repolarization) in the heart. Historically, ECGs were performed by placing electrodes on the left and right arms and the left leg. The voltage between the right arm and the left leg is called the lead II potential and is an indicator of heart-muscle function.
shows an ECG of the lead II potential and a graph of other major events during the cardiac cycle. The major features are labeled P, Q, R, S, and T. The P wave is generated by the depolarization and contraction of the atria as they pump blood into the ventricles. The QRS complex has a characteristic shape and time span, and is created by the depolarization of the ventricles as they pump blood to the body. The lead II QRS signal also masks the repolarization of the atria. Finally, the T wave is generated by the repolarization of the ventricles and is followed by the P wave in the next heartbeat. Arterial blood pressure varies with each part of the heartbeat, with systolic (maximum) pressure occurring closely after the QRS complex, signaling the contraction of the ventricles.
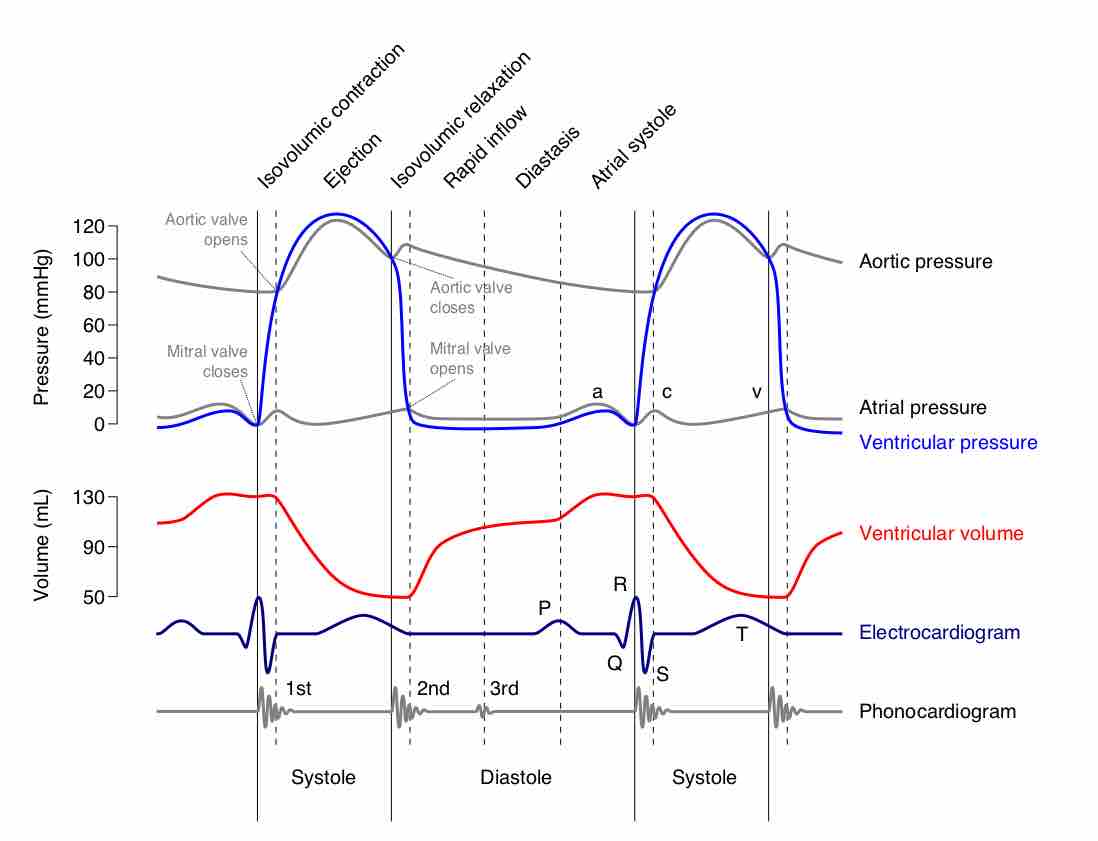
ECG Curve
A normal ECG curve synchronized with other major events during the cardiac cycle.