In the photoelectric effect, electrons are emitted from matter (metals and non-metallic solids, liquids, or gases) as a consequence of their absorption of energy from electromagnetic radiation of high frequency (short wavelength), such as ultraviolet radiation. Electrons emitted in this manner may be referred to as photoelectrons. This phenomenon was first observed by Heinrich Hertz in 1887.
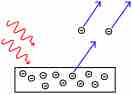
The Photoelectric Effect
Electrons are emitted from matter by absorbed light.
The photoelectric effect has been demonstrated using light with energies from a few electronvolts (eV) to over 1 MeV in high atomic number elements. Study of the photoelectric effect led to an improved understanding of quantum mechanics as well as an appreciation of the wave-particle duality of light. It also led to Max Planck's discovery of quanta (E=h
Planck's constant, h, is also known as "the quantum of action." It is a subatomic-scale constant and is one of the smallest constants used in physics. Other phenomena where light affects the movement of electric charges include the photoconductive effect (also known as photoconductivity or photoresistivity), the photovoltaic effect, and the photoelectrochemical effect.
Emission Mechanism
All atoms have their electrons in orbitals with well-defined energy levels. When electromagnetic radiation interacts with an atom, it can excite the electron to a higher energy level, which can then fall back down, returning to the ground state. However, if the energy of the light is such that the electron is excited above energy levels associated with the atom, the electron can actually break free from the atom leading to ionization of the atom. This, in essence, is the photoelectric effect.
The photons of a beam of light have a characteristic energy proportional to the frequency of the light. In the photoemission process, if an electron within some material absorbs the energy of one photon and acquires more energy than the work function of the material (the electron binding energy), it is ejected. If the photon energy is too low, the electron is unable to escape the material. Increasing the intensity of the light increases the number of photons in the beam of light and thus increases the number of electrons excited but does not increase the energy that each electron possesses. The energy of the emitted electrons does not depend on the intensity of the incoming light (the number of photons), only on the energy or frequency of the individual photons. It is strictly an interaction between the incident photon and the outermost electron.
Electrons can absorb energy from photons when irradiated, but they usually follow an all-or-nothing principle. Typically, one photon is either energetic enough to cause emission of an electron or the energy is lost as the atom returns back to the ground state. If excess photon energy is absorbed, some of the energy liberates the electron from the atom and the rest contributes to the electron's kinetic energy as a free particle.
Experimental Observations of Photoelectric Emission
For a given metal, there exists a certain minimum frequency of incident radiation below which no photoelectrons are emitted. This frequency is called the threshold frequency. Increasing the frequency of the incident beam and keeping the number of incident photons fixed (resulting in a proportionate increase in energy) increases the maximum kinetic energy of the photoelectrons emitted. The number of electrons emitted also changes because the probability that each impacting photon results in an emitted electron is a function of the photon energy. However, if just the intensity of the incident radiation is increased, there is no effect on the kinetic energies of the photoelectrons.
For a given metal and frequency of incident radiation, the rate at which photoelectrons are ejected is directly proportional to the intensity of the incident light. An increase in the intensity of the incident beam (keeping the frequency fixed) increases the magnitude of the photoelectric current, though the stopping voltage remains the same. The time lag between the incidence of radiation and the emission of a photoelectron is very small, less than 10−9 second, and is unaffected by intensity changes.
Mathematical Description
The maximum kinetic energy of an ejected electron is given by
where h is the Planck constant (6.626 x 10-34 m2kg/s) and f is the frequency of the incident photon. The term
The work function satisfies
where f0 is the threshold frequency for the metal. The maximum kinetic energy of an ejected electron is then
Kinetic energy must be positive for ejection to take place, so we must have f > f0 for the photoelectric effect to occur.
Photomultipliers
Photomultipliers are extremely light-sensitive vacuum tubes with a photocathode coated onto part (an end or side) of the inside of the envelope. The photocathode contains combinations of materials, such as caesium, rubidium, and antimony, specially selected to provide a low work function, so when illuminated by even very low levels of light, the photocathode readily releases electrons. By means of a series of electrodes (dynodes) at ever-higher potentials, these electrons are accelerated and substantially increased in number through secondary emission to provide a readily detectable output current. Photomultipliers are still commonly used wherever low levels of light must be detected.