Transduction of Light
The rods and cones are the site of transduction of light into a neural signal. Both rods and cones contain photopigments, which are pigments that undergo a chemical change when they absorb light. In vertebrates, the main photopigment, rhodopsin, has two main parts: an opsin, which is a membrane protein (in the form of a cluster of α-helices that span the membrane); and retinal, a molecule that absorbs light . When light hits a photoreceptor, it causes a shape change in the retinal, altering its structure from a bent (cis) form of the molecule to its linear (trans) isomer. This isomerization of retinal activates the rhodopsin, starting a cascade of events that ends with the closing of Na+ channels in the membrane of the photoreceptor. Thus, unlike most other sensory neurons (which become depolarized by exposure to a stimulus), visual receptors become hyperpolarized and are driven away from the threshold .
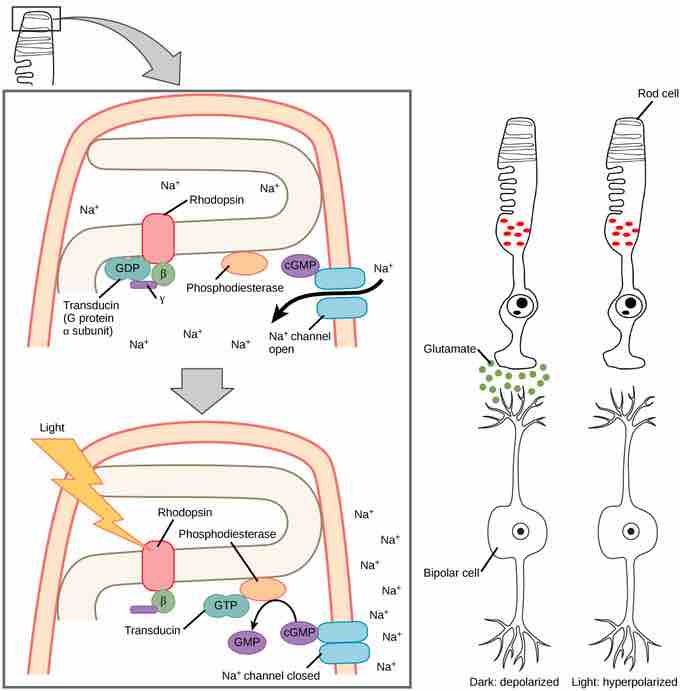
Hyperpolarized visual receptors
When light strikes rhodopsin, the G-protein transducin is activated, which in turn activates phosphodiesterase. Phosphodiesterase converts cGMP to GMP, thereby closing sodium channels. As a result, the membrane becomes hyperpolarized. The hyperpolarized membrane does not release glutamate to the bipolar cell.
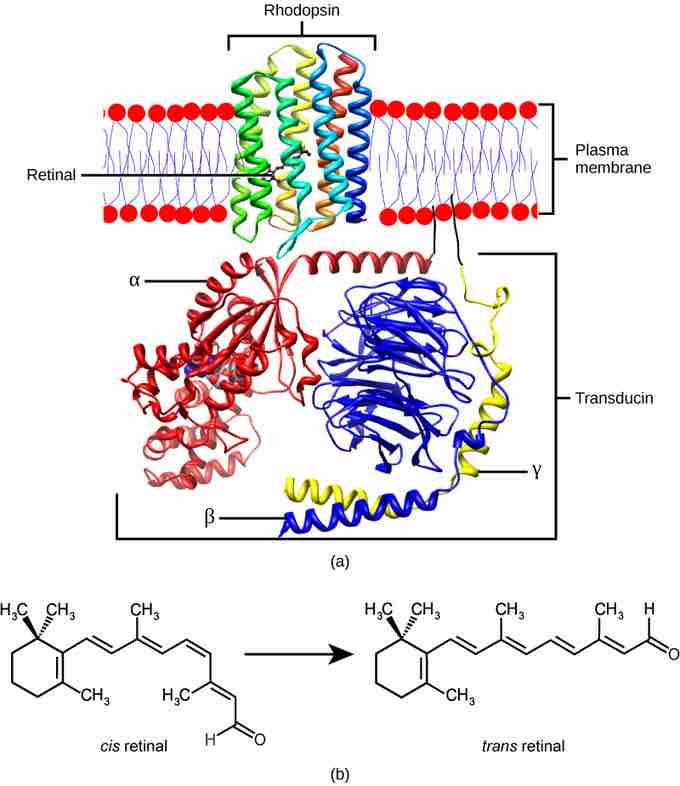
Rhodopsin
(a) Rhodopsin, the photoreceptor in vertebrates, has two parts: the trans-membrane protein opsin and retinal. When light strikes the retinal, it changes shape from (b) a cis to a trans form. The signal is passed to a G-protein called transducin, triggering a series of downstream events.
Trichromatic Coding
There are three types of cones (with different photopsins) that differ in the wavelength to which they are most responsive . Some cones are maximally responsive to short light waves of 420 nm; they are called S cones ("S" for "short"). Other cones (M cones, for "medium") respond maximally to waves of 530 nm. A third group (L cones, or "long" cones) responds maximally to light of longer wavelengths at 560 nm. With only one type of cone, color vision would not be possible; a two-cone (dichromatic) system has limitations. Primates use a three-cone (trichromatic) system, resulting in full color vision.
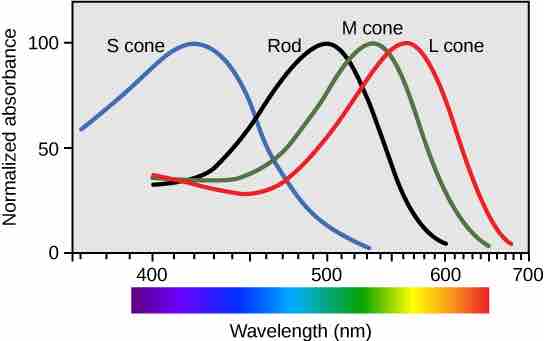
Rod and cone cells
Human rod cells and the different types of cone cells each have an optimal wavelength. However, there is considerable overlap in the wavelengths of light detected.
The color we perceive is a result of the ratio of activity of our three types of cones. The colors of the visual spectrum, running from long-wavelength light to short are:
- red (700 nm)
- orange (600 nm)
- yellow (565 nm)
- green (497 nm)
- blue (470 nm)
- indigo (450 nm)
- violet (425 nm).
Humans have very sensitive perception of color and can distinguish about 500 levels of brightness, 200 different hues, and 20 steps of saturation; in all, about 2 million distinct colors.
Retinal Processing
Visual signals leave the cones and rods, travel to the bipolar cells, and then to ganglion cells. A large degree of processing of visual information occurs in the retina itself, before visual information is sent to the brain.
Photoreceptors in the retina continuously undergo tonic activity. That is, they are always slightly active even when not stimulated by light. In neurons that exhibit tonic activity, the absence of stimuli maintains a firing rate at an equilibrium; while some stimuli increase firing rate from the baseline, other stimuli decrease firing rate. In the absence of light, the bipolar neurons that connect rods and cones to ganglion cells are continuously and actively inhibited by the rods and cones. Exposure of the retina to light hyperpolarizes the rods and cones, removing the inhibition of their bipolar cells. The now-active bipolar cells in turn stimulate the ganglion cells, which send action potentials along their axons (which leave the eye as the optic nerve). Thus, the visual system relies on changein retinal activity, rather than the absence or presence of activity, to encode visual signals for the brain. Sometimes horizontal cells carry signals from one rod or cone to other photoreceptors and to several bipolar cells. When a rod or cone stimulates a horizontal cell, the horizontal cell inhibits more-distant photoreceptors and bipolar cells, creating lateral inhibition. This inhibition sharpens edges and enhances contrast in the images by making regions receiving light appear lighter and dark surroundings appear darker. Amacrine cells can distribute information from one bipolar cell to many ganglion cells.